Picking good problems is one of the hardest parts of science. At some point in every scientist’s career, you achieve enough scientific maturity that you could study pretty much whatever you want. Given enough time, resources, and self-study, a mature scientist can in principle work on essentially anything you can imagine that doesn’t violate the laws of physics. The challenge becomes discerning which of many possible projects is the best use of your time.
There are many schools of thought on how to pick problems. During graduate school, my advisor Kevin often told me not to bother working on problems that will probably be solved without my help. Instead, he usually encouraged me to pursue ideas, approaches, or questions that I’m uniquely well suited to make a dent in. I expanded on this framework, and came up with some of my own rules of thumb for discerning amongst project ideas. One of them was to always have a story for why the problem you’re trying to solve will be easy for you, but hard for everyone else. Science is a lot of work. If you don’t have an angle, you’re just going up against the same brick wall as everyone else, and probably something else is more worth your time.
In the case of the qtRNA project, I had two stories:
I had access to super-rapid evolution techniques that previous researchers didn’t. In particular, I was very inspired by a paper in which they had evolved the ribosome to work better with four-base codons. They did so using traditional techniques that pale in comparison to the evolution technique I wanted to use, PACE, which pretty much blows everything else out of the water. (Prior work involved screening a library of 109; PACE screens a library of 109 to 1012 in just 20 minutes.) In Erika Update #3 (linked below) I talk about the first steps toward applying PACE to engineering better quadruplet codon translation.
I had access to high-throughput evolution techniques that previous researchers didn’t. The previous paradigm was one-PhD-one-engineered-protein. I had just built a robotic system that let me evolve ~100 proteins at once. This would let me tackle engineering problems that were probably impossible for other people. In the qtRNA project, I’m going after a system that has 20+ proteins.
Note that my taste differs from many scientists. People often follow the opposite approach: work on trendy research topics that have already proved themselves to be flashy and publishable. This was what the entire CRISPR era was about. Good work gets done this way too, although it puts you at greater risk of being scooped, and seems to just in general not be my style.
Erika Update #3
Here’s Erika Update #3 - 2018 3 17 - S UAGA Mag phage enrichment
I previously had created a reporter that will glow when my tRNAs work well. In this update, I created a new reporter that produces bacteriophage when my tRNAs work well. This is the first step toward using Phage-Assisted Continuous Evolution (PACE) to evolve these tRNAs at blazing fast speeds - I’m winding up to evolution. In the multi-color figure at the top of the third page, I show that I’ve successfully tied phage production to the ability of the phage to decode a four-base codon using a tRNA. I even take a stab in this update at adding one more level of complexity: having four-base codons be translated through an orthogonal ribosome (just like the paper that inspired this project!), but this part doesn’t work yet.
On the first page, I acknowledge that my problems with tRNA toxicity are becoming more “ominous.” Some tRNAs are just fine, others make bacteria very sick. So far I can’t see any pattern in which ones are which, and it’s becoming an impediment to progress because I can’t gather data when my bacteria are dead. This is the real beginning of the Surprise! It’s toxic theme.
Where’d it end up?
None of these graphs made it directly into a paper, although the fact that a phage bearing the un-evolved Ser-UAGA tRNA doesn’t plaque on pIII-29-UAGA did make it into Supplementary Figure 4b of Paper #1, which has a little annotation that says “does not plaque” 🤣.
Vocabulary highlight: “Phage”
One of the key techniques I’m working with is Phage Assisted Continuous Evolution (PACE). Before we get to the rest of the acronym in a future Update, let’s just understand what a phage is.
Phage is short for “bacteriophage”, which means “bacteria eater.” Basically, a phage is a virus that infects bacteria. It can’t infect you! The particular type of M13 phage I was working with infects bacteria by interacting with proteins that aren’t even in the human genome.
In a bit more detail, the M13 bacteriophage is essentially a bacterial sexually transmitted disease. Although bacteria don’t have sex in the traditional sense, they are able to exchange genetic information by expressing a pilus (a bacteria penis) and using the pilus to exchange bits of DNA with other bacteria. The M13 bacteriophage infects bacteria through the pilus.
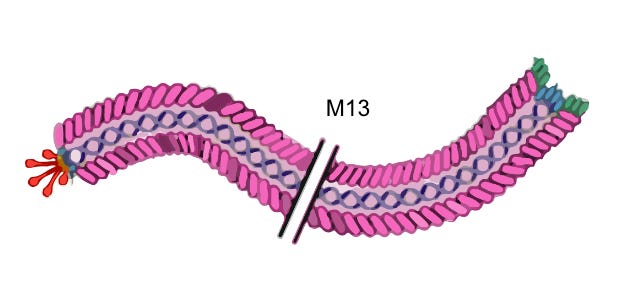
Viruses come in all shapes and sizes. You may be most familiar with viruses that look like COVID (circular with bits sticking out) or like spiders (geometric with legs). M13 has its own unique filamentous shape, which means it’s a super long skinny worm-looking virus that has the virus genome in the middle and ‘tail fibers’ at the ends. Unlike a lot of other viruses, M13 doesn’t actually kill bacteria when it infects. Instead of filling the bacteria with baby viruses and then exploding the cell (a lytic life cycle), M13 hijacks the bacteria to steadily extrude baby viruses into the media that bud out of the bacterial membrane like hair (a chronic life cycle).
And to orient you
I think pretty much everyone can get something out of reading these blog posts + updates. Here’s some additional notes customized for you, depending on your interests + background:
You’re a non-scientist: In the bullet points at the bottom of the first page, we can witness Erika becoming increasingly bemused by these tRNAs. Some — but not all! — of them are very toxic. They just kill bacteria, which makes it hard to do science! This is hugely inconvenient. There are many sensible patterns for which ones should be toxic… and none of those patterns appear to fit the data. This is the beginning of the toxicity mystery, which takes quite a lot more elbow grease to overcome.
You’re a student doing a PhD/undergrad research/etc: The graph on the top of page 3 is very satisfying. It has a negative (purple) and positive (orange) control. The conditions I’m testing (blue, red, green) fall in between, just like I’d expect, and are ordered in a way that makes sense. The best part is that the y-axis is a log scale! All of these things together mean that this is data you can feel quite secure interpreting.
You have ideas for reforming publishing: The literature implies that you can pretty much put any anticodon into a serine tRNA — after all, SerRS doesn’t recognize the anticodon loop at all. Yet when I tried new anticodons, some of them are extremely toxic!? I’m sure someone has observed this before. It’s truly insane that it was never published and I had to re-discover this roadblock myself.
You’re a fellow PACE nerd: Here’s an important tip for getting a new PACE circuit to work: expression of pIII in E. coli causes the F pilus to retract, making the bacteria uninfectable. If your circuit expresses too much pIII by default nothing whatsoever will work because the phage can’t even infect the bacteria! It’s important to confirm that wildtype M13 phage can form plaques in E. coli carrying your accessory plasmid.
You’re a fellow genetic code expansion nerd: I talk a bit at the end about how residue 29 and residue 34 of pIII appear to have different propensities for frameshifting.
Want more?
If you want to follow along with this project, you can get updates by signing up through substack, or following me on linkedin or twitter.
If you have ideas for what I should cover in the blog post, suggestions for vocabulary to define, questions about the science, or other comments, please do comment on substack or reach out by twitter DM - I’d love to hear from you!